How Is Climate Change Affecting Ocean Waters and Ecosystems?
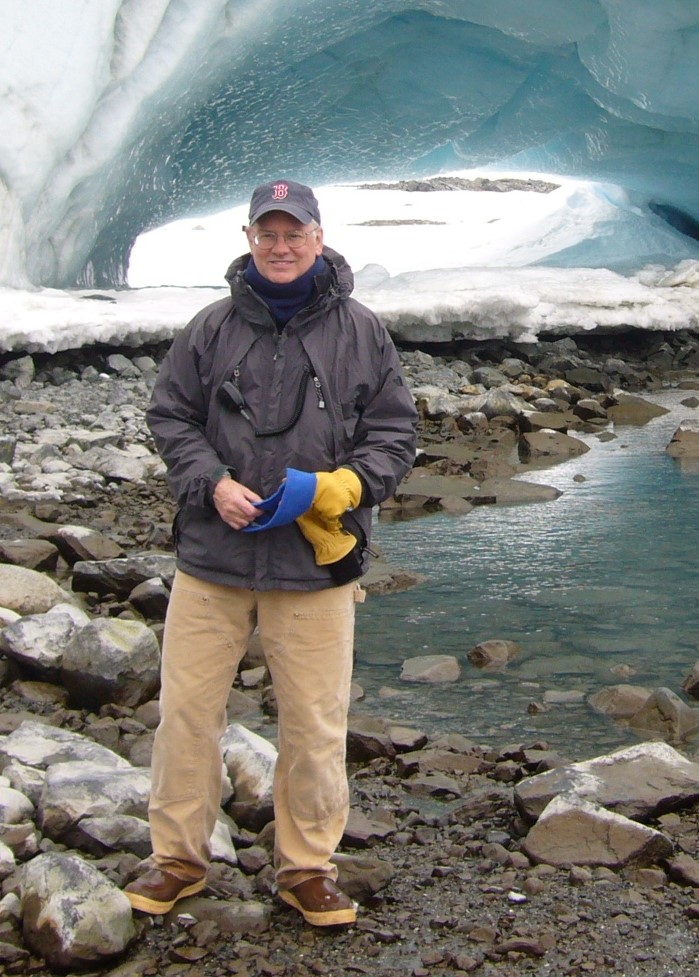
Biological oceanographer Hugh Ducklow describes decades of work in far-flung places to understand the evolving ecology of the oceans. The picture is not always clear.
|AUGUST 29, 2022
Biological oceanographer Hugh Ducklow studies the marine food web, and how it interacts with the physical properties of the oceans. Much of his work is through the U.S. Long Term Ecological Research Program (LTER), in which researchers have for decades investigated trends across 28 land and marine regions in the United States, along with a few sites elsewhere. In addition to the open ocean, studies encompass deserts, coasts, rivers, forests and grasslands. From 2012 to 2018, while based at Columbia University’s Lamont-Doherty Earth Observatory, Ducklow led the Palmer Station LTER site, the base for yearly cruises through 800 kilometers of icy waters off the Antarctic Peninsula.
To mark the 40th anniversary of the LTER program, researchers just published a series of articles on how climate change is affecting their sites. Ducklow led the section on open-ocean environments, which in addition to Antarctica spans waters off Alaska, California and the U.S. Northeast. We spoke with him about the work, his and colleagues’ observations, and prospects for the future.
Photo Credit:
Ducklow at a glacial ice cave near Palmer Station, Antarctica, 2006. The glacier collapsed and wasted away a year or two later. (Courtesy Hugh Ducklow)
Why should we care about what climate change does to the oceans?
Besides the fact that seafood constitutes the major protein source for about 3 billion people, the ocean soaks up a major amount of excess heat and human-generated carbon dioxide. Around 90 percent of all the excess heat produced by the greenhouse effect since the Industrial Revolution is in the ocean. The global ocean has also taken up about one quarter to one third of our carbon dioxide emissions. Both these processes keep air temperatures cooler than they would be otherwise. But they both come with costs. The ocean is warming as a result of added heat. The human-caused warming signal is even detectable in the deep Southern Ocean. Enhanced carbon dioxide uptake is causing ocean acidification. The ecological consequences of warming and acidification are just beginning to be understood, and the future capacity to continue to store heat and CO2 is not certain.
What are the some of the physical effects of climate on ocean waters, and where are we seeing them most strongly?
Like I said, the oceans are warming, but the warming and its effects are not uniform in space or time. Responses to climate change by the physical system are strongest and most evident right at the surface. This is important because heat and CO2 are exchanged there, and because phytoplankton grow there. Depending on winds, storms and currents, the surface layer will vary in depth from nearly zero in summer to over 1,000 meters in winter. Temperature affects the depth of the surface layer, and in the case of polar sites, so does sea ice. Near the poles in winter, there is little or no solar irradiation, and sea ice covers the ocean. In the spring, as the sun rises, the surface ocean warms and sea ice melts, adding freshwater to the surface. Warmer, fresher waters are less dense than cooler, saltier waters, and so the surface layer shallows.
Surface mixed layer depth is getting shallower at most sites in the LTER network—Palmer Antarctica, the Northeast U.S. continental shelf and the Northern Gulf of Alaska. However, no change is evident in the California Current, in spite of an unbroken record of observations since 1950 and warming water temperature.
What biological changes are taking place? Can we link them clearly to climate trends?
The depth of the ocean surface layer controls the rate of phytoplankton growth. When the surface layer is shallow, phytoplankton are retained in sunlight, but lack access to nutrients. When the surface layer is deep, phytoplankton can access nutrients, but sunlight is dim or absent. Trends in phytoplankton have been documented in some, but not all the LTER sites. Phytoplankton are the only organisms that can be detected by satellite, but trends in their abundance are not so clear as the physical changes I just described. Evidence of phytoplankton is increasing in Antarctica, as expected in a shallowing surface layer, but decreasing over the Northeast U.S. continental shelf, in spite of shallowing. No changes are evident at the other sites. Zooplankton show increasing trends in Antarctica, as expected from increasing phytoplankton. They are also increasing in the California Current system, even though phytoplankton aren’t.
Even though there are long records of changes in the California Current (70 years), Northeastern U.S. shelf (40 years) and Palmer Antarctica (30 years) it’s still difficult to say for certain that they’re caused by climate change. Numerical simulations of satellite imagery suggest about 50 years is the minimum time needed to attribute observed trends to climate change. Some changes may take a century or longer.
Are there things happening in Antarctica that distinguish it from the other regions?
One simple distinguishing feature of Arctic and Antarctic seas is that they’re covered by sea ice. But the duration and extent of ice cover are declining as the polar oceans warm. The life cycles of Arctic and Antarctic organisms such as krill and seabirds are attuned to seasonal ice cover, and may be disturbed as the cover decreases. Sea ice blocks sunlight, influencing the timing of phytoplankton blooms. Although sea ice is decreasing rapidly at both poles, the effects are uncertain. As sea ice declines, new, formerly ice-covered areas are opened up for phytoplankton growth, expanding the polar marine ecosystem. But as the cover disappears, its contribution of freshwater will decline and decrease the fresh layer at the ocean surface. The net impact for the future ecosystem isn’t clear.
Another distinguishing feature of Antarctic ecosystems appears to be the diversity and pace of ecological change. We assume that climate variability and change first affect physical properties and then the physical changes cause ecological responses. The ecological responses can be organized into those that start with phytoplankton at the base of the food web, that is, bottom-up responses; and those that affect the top predators like penguins with changes rippling down through the food web, or top-down responses. In Antarctica, we’re seeing changes in the climate and physical systems and throughout the food web, from diatoms to krill to penguins. These processes meet in the middle, converging on krill.
Have we been observing these sites long enough to get a good idea of where things are headed in the future?
How long is needed to know where ecosystems are headed depends on what changes you’re interested in. It’s easier to observe and document physical changes, because the system only consists of heat, salinity, currents and mixing—and because we have good instruments to make precision measurements of those variables. In contrast, dozens to hundreds of different measurements are needed to characterize variability in multispecies biological responses, and only a few can be sampled and measured remotely. With a few key exceptions, detecting changes for many groups of organisms still depends on individual scientists and students making simple, time-consuming and tedious one-by-one visual counts. These measurements are slowly becoming automated. Drones, ship-mounted acoustics, submersible digital video cameras and instrumented ocean gliders are beginning to make real-time, comprehensive views of the oceans. Sea ice cover and icebergs are still big obstacles to leaving instruments unattended over the winter, so a lot of measurements are restricted to ice-free summer months.
What have been some of the challenges in working off Antarctica?
There are the obvious challenges: planning work in a remote location—travel takes seven days door to door each way—and anticipating everything you might need. There’s storms, high seas, ice cover. We got stuck in the ice for two weeks in September 2001. Then supply-chain issues, personnel recruiting, and maintaining a high-quality time series of observations and measurements over decades. The work to prepare for next year literally starts before you depart for the ship this year. The project is not simply the time series, but living, evolving scientific research with wrong turns, blind alleys and unexpected discoveries. In spite of the challenges, it’s a beautiful and exciting place to work.